Exploring the limits of quantum theory for arbitrarily massive objects is a fascinating and ambitious endeavor at the interface of quantum mechanics and general relativity. While most quantum experiments have involved relatively small particles (like electrons and photons), there is growing interest in examining how quantum mechanics applies to larger, more massive systems.
Use a highly sophisticated optomechanical setup where a large mechanical oscillator (e.g., a vibrating membrane or a large mass-spring system) can be prepared in a coherent superposition of different vibrational states.
The mass of the object should be on the order of milligrams to grams, significantly larger than prior experiments typically performed with smaller quantum systems.
Prepare a massive object in a quantum state using techniques such as laser cooling or electromagnetic trapping to minimize thermal noise and create a ground state for precise manipulation.
Employ a coherent light source to implement a Quantum State Control protocol, manipulating the object into a superposition of two distinct vibrational modes. For instance, you could create a superposition of the object being oscillated at two different frequencies.
Conduct the experiment in a highly isolated environment to minimize external interactions. Employ a vacuum chamber, magnetic shielding, and maybe even gravitational shielding to reduce interference from environmental factors.
Monitor the object’s state over time to observe how quickly decoherence occurs. Use sensitive measurements (like interferometry or photodetection) to track the evolution of the wavefunction and identify when it collapses to a definite state.
Gradually increase the mass of the oscillator in subsequent experiments to map out the transition from quantum behavior to classical behavior. This could involve using different materials or configurations to assess whether certain mass thresholds influence decoherence.
Quantum-to-Classical Transition:
Source
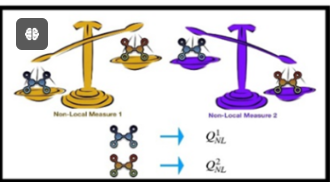